Understanding how the global-mean precipitation rate will change in response to a climate forcing is a useful thing to know. We have strong evidence that the hydrologic cycle will become more intense in response to CO2, but quantifying what drives this change is a bit more complicated, and can be understood from a few different perspectives. This paper takes a unique approach that really helped my understanding of the problem.
The approach of this paper is different than many previous papers that focus on the energy budget at the surface or the top of the atmosphere (TOA). Instead, the authors use the energy budget of the entire atmosphere itself. The authors show how the global-mean precipitation is constrained by the atmospheric cooling.
In the above equation for the global energy budget, latent and sensible heat fluxes (LE and SH) transfer energy from the surface to the atmosphere. On long time scales, evaporation (i.e LE) balances precipitation. Sensible heat flux is the turbulent transfer of heat, but it is generally much smaller than the latent or radiative energy flux. So, to first order, the main balance from this perspective is between radiation and precipitation. Radiation generally cools the atmosphere and precipitation generally warms it.
The novel aspect of this perspective that I had not appreciated before is how the atmosphere radiates out to space AS WELL AS down to the surface. This cooling to the surface can actually be a pretty large source of cooling.To illustrate how important this is, the authors put this really informative table from some idealized radiative calculations.
For example, the first row describes a uniform warming of the atmosphere, which increases the cooling to space by 2.3 W m2, but also increases downward emission to the surface by 3.3 W m2. There are plenty of other interesting examples here that I found useful to work through in my head.
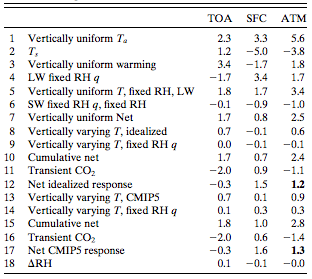
TABLE 2. Clear-sky radiative response to various … changes at the TOA, [SFC, and ATM], calculated with Fu and Liou (1992) column radiation code. Signs are chosen so that a positive response contributes to increasing precipitation: increased cooling is positive for the atmospheric column, upwelling is positive at the TOA, and downwelling is positive at the surface. Note that the atmospheric cooling is the sum of net downwelling surface radiative flux and net upwelling TOA radiative flux: ATM = SFC + TOA…
“The wavelengths of water vapor continuum absorption are most important in determining surface emission, while upper tropospheric emission is determined by the wavelengths of rotation and vibrational bands (Mitchell et al. 1987; Inamdar et al. 2004).”
The authors go on to compare their calculations to CMIP5 model output, and the idealized calculations do a good job predicting the result of the models. They also do some idealized calculations of cloud effects which I found interesting. The shortwave effect of low clouds is often discussed in terms of a feedback mechanism, however they show here that the LW effect easily dominates the energy budget. Their idealized increase of high cloud amount has the strongest impact on global precipitation (see their table 4 below).
“Here we encounter one of the great advantages of the atmospheric energy budget perspective over the surface perspective: reflection of SW by clouds has little effect on the atmospheric energy budget. There is a modest change in SW absorption when clouds are present, but this is far overwhelmed by the clouds’ effects in the LW.”
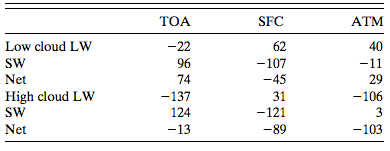
TABLE 4. Radiative response (W m-2) to idealized clouds. As in previous tables, signs are chosen so that increased atmospheric cooling is positive.
This quote below was something I didn’t actually know. I always feel like an idiot when I read “It is well know…” followed by something I didn’t know…
“It is well known that the lapse rate and water vapor feedbacks compensate each other at the TOA (e.g., Cess 1975;Zhang et al. 1994; Soden and Held 2006; Held and Shell 2012).”
One last note, the energy budget framework can also be used to understand the consequences of geo-engineering scenarios that block incoming solar radiation to compensate the warming by CO2:
McCusker et al. (2012) performed an experiment in which global-mean surface temperature was held constant by increasing CO2 while simultaneously increasing sulfate aerosol… to compensate. …precipitation decreased steadily as CO2 and stratospheric sulfate aerosol increased….The CO2 forcing caused the atmospheric cooling rate to decrease. The temperature did not change, so the surface temperature-dependent responses of atmospheric radiative cooling also did not change. The sulfate aerosol acts by reflecting SW …, but this has little effect on the atmospheric energy budget and precipitation. The CO2 forcing can be viewed as directly suppressing the precipitation in this experiment, causing precipitation to decrease even without surface temperature change.
in your expert opinion, do CO2 and H2O, lose their vibrational energy (greenhouse effect) at subzero temperature of high altitude – 40,000 to 100,000 feet? If they do, would that energy eventually be emitted into space?
Thanks
That’s a bit of an odd question. All molecules are gaining or losing energy continuously, it never just stops for specific conditions. Also, the planet is constantly emitting to space, and we can’t track exactly how energy flows through the system. The greenhouse effect isn’t something that only operates at certain elevations. It’s better to think of the greenhouse effect as the net radiative impact of an atmospheric column that includes molecules that absorb and emit in the same wavelengths that are being emitted by the surface of the planet. In our case this we use the term “longwave” to refer to this part of the electromagnetic spectrum. Hopefully that answers your question?
Thanks for reply. I understand that absorption and emittance of energy by greenhouse gases is an ongoing thing in lower atmosphere. But in the case of water molecules once they have been converted to liquid or ice in high altitude, they would lose their vibrational energy and since the air is thin up there, eventually all that energy is lost into space. The same should hold true for CO2. I am assuming that water upon changing phase to vapor at lower altitude, along with CO2 would absorb IR energy and release it back into space.
In the mind of this 87-year-old chemist, that is the only logical explanation for the survival of our planet 450 million years ago when CO2 concentration in the atmosphere was in excess of 7,000 ppm. That was when mollusks appeared on earth and gulped down all that CO2 in the process of making their mantel; 44% CO2
I think you mean that when water changes to ice it would lose its greenhouse effect potency, but that’s not really true. Thin ice clouds in the upper troposphere actually have quite a large long wave radiative impact. And CO2 doesn’t really undergo phase changes in the atmosphere as far as I know. Maybe there’s frozen co2 in the upper stratosphere, but I doubt it.
It is my understanding that the greenhouse effect is due to certain wavelength of infrared radiation having precisely the right energy to interact with appropriate molecules in the earth atmosphere and increase their temperature. These molecules then reradiate the heat to their surroundings. That is what happens to IR energy of water vapor when it changes phase from gas to water and ice in high altitude. Although since the temperature up there is still above zero Kelvin, certain portion of the IR could remain with the ice crystal molecules and eventually reflected back into atmosphere; revised in 2004 by Trenberth from 77 W/m2 to 79 W/m2.
In nutshell, the greenhouse gases from the beginning absorb the proper IR energy in lower atmosphere and lose it in cold high altitude. That is the only explanation for plants and animal not being vanished 450 million years ago when CO2 in the atmosphere was higher than 7,000 ppm
That’s not an accurate characterization of the greenhouse effect, and I don’t think it can explain how life survived in an atmosphere that was more opaque to infrared radiation.